The nevado Ampay, which is part of the Ampay National Sanctuary: guards the city of Abancay, is capped by a substantial glacier and year-round snow. Ampay National Sanctuary contains nevado Ampay peaks, 5,225 meters above sea level (masl), lake Usphaqocha, lake Ankasqocha and lake Q’elloqaqa, making it a tourist attraction, however, low socio-economic communities near the Sanctuary collect firewood for their daily activities releasing Black Carbon (BC) in the vicinity of the glacier.
The objective of the investigation was to determine the amount of BC that decreases the albedo of the surface of the Ampay glacier, and to evaluate the loss of mass of the glacier and the temporary variability of the BC in the period January-December 2017.
Glaciers in the Andes of Peru provide important environmental and economic services. Roughly 70% of all tropical glaciers are located in Peru. The most extensive glacial range in Peru is the Cordillera Blanca, which hosts nearly a quarter of all tropical glaciers [1]. South America is home to 99% of the worlds tropical glaciers [2]. In the last years of the twentieth century, climate change has caused significant changes in the systems of the high Andean areas, highlighting the negative impact on tropical glaciers, called glacial retreat [3].
BC is a type of short-term climate pollutant, its global warming potential is estimated at up to 5,000 times more than carbon dioxide (CO2) [4]. It is positioned as the second or third climate controller behind CO2[5]. This problem has an impact on a global scale [6]. Unlike volatile organic compounds that form a single carbon structure component that is transparent to the human eye, BC absorbs visible light and, therefore, is responsible for decreasing visibility in the air.
BC in a glacier implies the reduction of the albedo (reflection of light), which means a greater melting of the snow ice, due to this a greater amount of short-wave solar radiation is absorbed and transferred in the form of heat to the glacier. The objective of the investigation was to determine the amount of BC that decreases the albedo of the surface of the Ampay glacier, and to evaluate the loss of mass of the glacier and the temporary variation of the BC in the period January-December 2017.
For compounds that are emitted into the atmosphere, BC is the main substance that absorbs solar radiation [7]. By absorbing solar radiation, pollution decreases the incidence of radiation on the surface, but the net effect on the earth-atmosphere system (known as radiative forcing) represents an increase in surface and atmospheric temperatures [8], in addition to changes in air temperature [9]. Dust and BC deposited in snow could be a potentially important factor leading to loss of glacial mass [10].
The powder and the BC called Light-Absorbing Particles (LAP) reduce the albedo of the snow and cause a greater absorption of solar radiation, which leads to an increase in the rate of fusion and sublimation [11].
The dust comes from natural sources (suspension of mineral dust and volcanic eruptions) and anthropic (processes of extraction, construction and demolition), in the same way that the BC comes from natural sources (natural forest fires) and anthropic (burning to open sky and road traffic), process where there is incomplete combustion [12], the main sources of BC emissions [13]. They are in the transport sector powered by Diesel [14], situation that is also observed by the irresponsible burning of biomass [15]. However, households located in the rural area are the most affected [16], all this huge problem on a large scale has been particularly affecting developing countries [17].
In Latin America, the most important source of BC is open burning with 70%, followed by burning fossil fuels in the land transport sector with 14% [18].
In parallel with the glacier retreat, climate in the tropical Andes has changed significantly over the past 50-60 years. Temperature in the Andes has increased by approximately 0.1 °C/decade, with only two of the last 20 years being below the 1961-90 average [19].
The tropical glaciers are located in the highest areas of the belt that surrounds the terrestrial globe, which extends from the height of the central zone of Mexico to the extreme north of Argentina, and which is known as a torrid or tropical zone. These glaciers are the largest reserves of fresh water frozen in the earth after the poles [20].
According to geologist specialized in glaciers, Lonney Thompson, who studies this phenomenon in Peru since 1974, the melting will seriously affect the water supply (for 70% of the Andean countrys population) and the climate in this region of South America [3].
The anthropogenic impact adds particulate material that goes towards the glaciers and increases the levels of pollution in the atmosphere. In Peru, the Cordillera Blanca has suffered a 30% decrease between 1970 and 2003, the Cordillera Vilcanota 33% between 1970 and 2009 and the Cordillera Huaytapallana 55% between 1970 and 2009 [3].
Glaciers in the tropical Andes have receded by approximately 30% since the 1970s and area loss rates increased substantially in the first decade of the new millennium showing a change in surface air [1]. The temperature has increased by 0.10 °C per decade during the last 70 years in the tropical Andes according to the measurements of 279 stations between 1° N and 23° S.
The use of climate models has shown that the tropical regions of the glacier regions (more than 5,000 m) could experience a temperature increase of 4 to 5 °C between 2000 and 2100 [21].
Forest fires also emit by products that are toxic to the environment, such as hydrogen cyanide, organic carbon and BC, among others [22]. Smoke inhalation deaths from wildfires are 350,000 per year worldwide [23].
Large-scale wildfires have increased in recent years [24], and evidence also suggests that mega-fires are positive feedbacks to climate change through carbon emissions. Ecosystem services will be affected, especially carbon sequestration capacity and water yield [25].
Given that many impacts are temporally variable throughout the year, it is valuable to collect measurements throughout a calendar year. Year-round measurements are valuable for national policy decisions to mitigate the impacts of climate change if possible.
Therefore, BC has an important and unique role in the earths climate system because it absorbs solar radiation, influences the formation processes and dynamics of clouds and significantly alters the snow melting process and ice covers, the great uncertainty in the estimation of its short-term (20 years) and long-term (100 years) effects, reflecting the great challenges to understand and quantify its effects.
The water that comes from the glacier in the future could generate repercussions for the inhabitants due to the accelerated decrease due to climatic actions and concentrations of BC. Atmospheric pollutants, such as BC particles, can be deposited on glacial surfaces by precipitation processes or by ‘dry deposition’. BC on the surface of glaciers increases the rate of fusion by light absorption. That would be reflected differently [26], Although studies have shown that the impact of BC albedo on glaciers is significant, the actual extent of BC deposition on tropical glaciers is still under investigation. BC, while promoting the melting of snow and ice, also affects the decrease in rainfall [27].
The research process shows that BC is a short-lived aerosol and the study conditions facilitate the results regarding glacial melting in annual periods from January to December, obtaining laboratory results for the first time in an Ampay glacier investigation, considering that it is an area protected by the government of Peru as a National Sanctuary, therefore, the importance increases. The results obtained annually show greater accumulation of informative data for similar studies in the Ampay glacier.
The annual evaluation allows showing the variation of the concentration and its relation with the weather conditions around the Ampay glacier.
The Light Absorption Heating Method (LAHM) technique developed by Carl G. Schmitt has been applied, which consists of Quartz, this is analyzed in a laboratory where it is subjected to filter heating by exposing them to an artificial light source where changes in filter heating are observed to determine the light absorption capacity. This technique has been used for more than 3 years in the Cordillera Blanca of Peru for the sampling of BC by the American Climber Science Program using a method known as LAHM, obtaining a minimum of 2 and a maximum of 80 ng/g of effective BC as a unit of measurement (ACSP) [28].
The samples were collected at different altitudes to a depth not greater than 3.0 cm, once the collection has been completed, they were stored in a cooler for subsequent filtering. The ziploc bags are heated to a temperature of approximately 30 to 40 °C, this in order to melt quickly, then using a 60 ml syringe of water, 60 ml was withdrawn, then slowly pumping the water through the filter, an approximately 30 to 60 seconds to pass the 60 ml through the filter.
It should be noted that the filter has been 25 mm with a porosity of 0.7 mm, then the number of syringes that depended on their saturation has been noted [28].
With this technique, the increase in temperature of the particles in a filter is measured by applying visible light to it.
Each filter is suspended with transparent plates and exposed to a visible light lamp under the filter, approximately 5 cm away, an infrared thermometer controlled by an Arduino processor is placed, which records the temperature every 0.25 seconds [28].
For the measurement of light-absorbing particles, each filter is exposed to five cycles of visible light. Each cycle is comprised of 3 stages of filter temperature measurement:
-
Filter reference temperature by recording measurements for 10 seconds;
-
Temperature during exposure to visible light source for 30 seconds;
-
Post temperature exposure to light source for 90 seconds.
Filter temperature rise results are calibrated to standards prepared with BC solutions of known concentrations. BC standard is passed through a filter in the same way as samples collected in the field of impurities in the snow (Figure 1). The LAHM excel calculation has been to 20% uncertainty (80% confidence) based on preliminary measurements according to Carl Schmitt who designed the instrument.
After the samples had been filtered, they were left to dry for about a day, placed in filter holders, the filters are placed in capsules designed for the coin collection for storage, the filters are removed from the coin capsules with tweezers, plastic and placed in the LAHM analyzer, then connected to a laptop that has previously installed ARDUINO software (Figure 3 and Table 1) and the script has been run, the output data has been stored in .txt files for further processing in LAHM spreadsheets [28] (Figure 3).
BC is one of the most important light-absorbing aerosol species in the atmosphere, which has the potential to affect regional climate due to its absorption and consequent atmospheric warming. Despite being a smaller fraction of the composite aerosol system, the BC can compensate for the radiative cooling resulting from the dispersion of solar radiation by sulphate and other nonabsorbent aerosols [29].
BC of the biomass and the combustion of fossil fuels alter the physical and chemical properties of the atmosphere and albedo of the snow, however, little is known about its history of emissions or deposits [30].
BC emissions are the second largest contributor to current global warming after CO2 emissions [31].
They calculate that the direct BC climate pressure of 0.9 W/m2, which is up to 55% of the CO2 pressure, is greater than the pressure attributable to other greenhouse gases such as methane (CH4), chlorofluorocarbons (CFC), nitrous oxide (N2O) or ozone (O3) of the troposphere.
If the precursor sources of CH4 and O3 are reduced in the future, the change in climate forcing by non-CO2 greenhouse gases in the next 50 years could be almost zero, combined with a reduction in carbon emissions [32].
Other scientists estimate that the direct pressure of BC is between 0.2 and 0.6 W/m2 with different ranges due to uncertainties. This compares with the Intergovernmental Panel on Climate Change (IPCC) climate pressure estimates of 1.66 W/m2 for CO2 and 0.48 W/m2 for CH4 and does not include the critical indirect effects of BC on the snow/ice albedo. These effects make BC pressure between two and three times more effective in increasing temperatures in the northern hemisphere and in the Arctic as equivalent CO2 pressure values.
Burning creates other pollutant emissions, such as particulate material in the form of soot or BC [32]. Taking into consideration that the variables to be analyzed have to be considered in the field studies and observations and, if possible, measured in the field, the design is prospective. In the case of the Ampay glacier, the method of analytical induction has been applied. The procedure consisted of collecting snow samples in several places of the glacier, after melting the samples, passed through quartz fiber filters (Figure 1 and Figure 2), demonstrating that they spend less than 80% and more often around of 95% of BC particles that are absorbed by the particles in the snow. 10 field trips have been made, has evaluated the presence of the pollutant, as well as the temporal variation of BC, which has been considerable in the months of September, October and November when temperatures increased.
Quartz filters with snow samples with BC
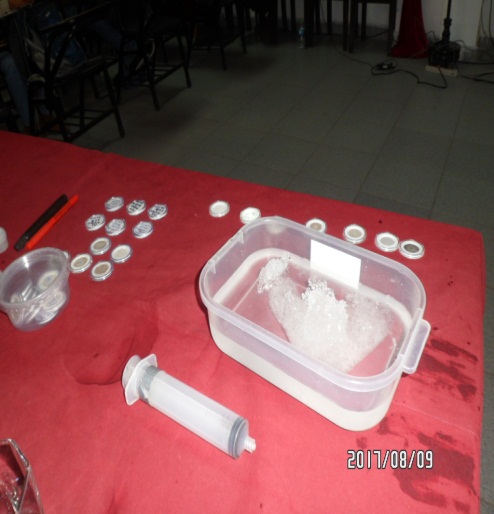
Determined snow and passing through the quartz fiber filters
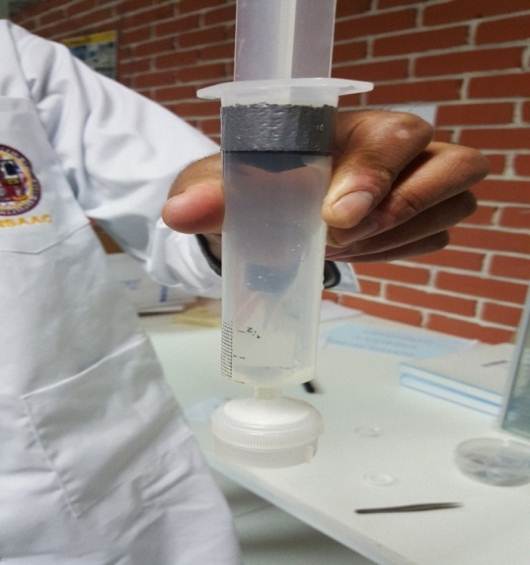
Snow samples were collected at various locations in the Ampay glacier, the snow melts and the liquid passes through quartz fiber filters.
The filters are analyzed using a thermal method that directly measures the capacity of the particles captured in the filter to convert visible light into thermal energy.
Filter analysis using LAHM
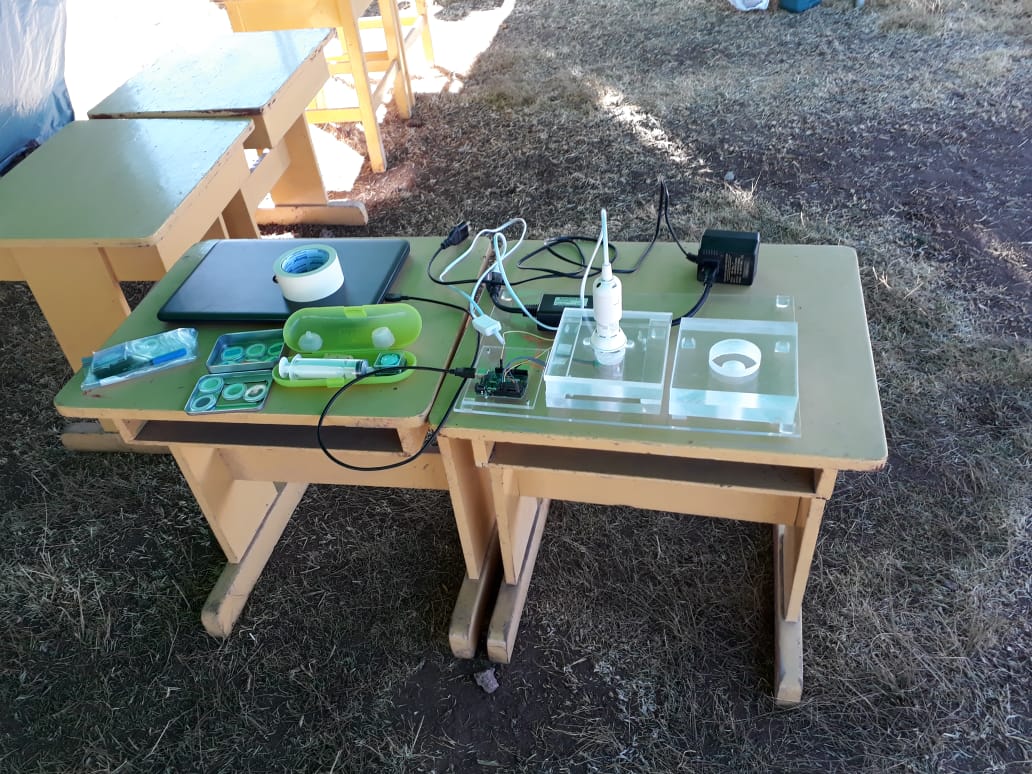
In the process, the instrument LAHM (Figure 3), finds mathematical correlation with the filters through the ARDUINO SOFT programming package, as shown in the Table 1.
Simulation results of the loss of snow albedo with BC using ARDUINO software
Total time [s] |
Time in cycle [s] |
Temperature [°C] |
Light on/off |
Cycle |
0.25 |
0.00 |
16.72 |
0 |
0 |
0.50 |
0.25 |
16.64 |
0 |
1 |
0.75 |
0.50 |
16.64 |
0 |
1 |
1.00 |
0.75 |
16.68 |
0 |
1 |
1.25 |
1.00 |
16.64 |
0 |
1 |
1.50 |
1.25 |
16.52 |
0 |
1 |
1.75 |
1.50 |
16.68 |
0 |
1 |
2.00 |
1.75 |
16.58 |
0 |
1 |
2.25 |
2.00 |
16.56 |
0 |
1 |
2.50 |
2.25 |
16.52 |
0 |
1 |
2.75 |
2.50 |
16.48 |
0 |
1 |
3.00 |
2.75 |
16.52 |
0 |
1 |
3.25 |
3.00 |
16.46 |
0 |
1 |
3.50 |
3.25 |
16.48 |
0 |
1 |
3.75 |
3.50 |
16.52 |
0 |
1 |
4.00 |
3.75 |
16.64 |
0 |
1 |
To understand this process, SNICAR software has been used, which simulates the loss of snow albedo with a certain amount of BC, determined according to the LAHM methodology between February to November 2017 (Figure 4).
This simulator provides hemispheric reflectance (albedo) of snow for unique combinations of impurity content (BC, dust, and volcanic ash), snow grain size, and characteristics of incident solar flux [33]. The simulator is a single-layer implementation of the Snow, Ice, and Aerosol Radiation Model (SNICAR) [34], using the two-stream radiative transfer solution [35].
Software SNICAR for sample simulation
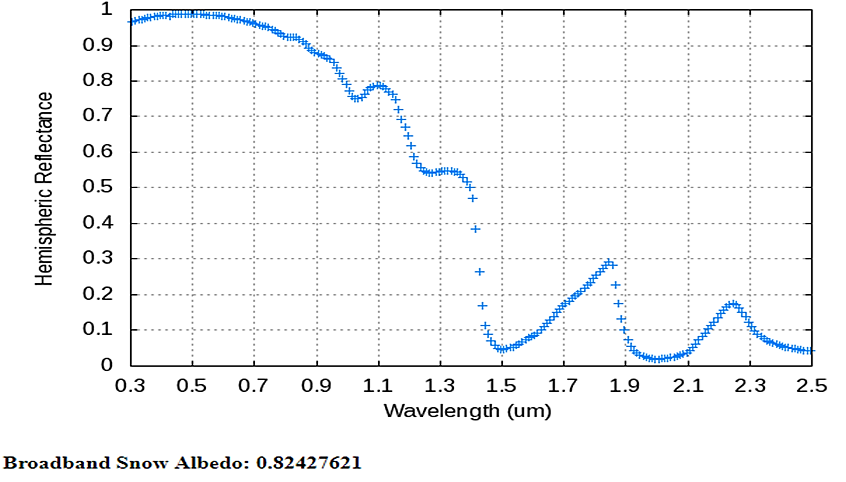
The Ampay glacier is part of the Cordillera de Vilcabamba, it is located in the region of Apurimac, province of Abancay, its maximum altitude is 5,235 amsl, and it is located geographically at the coordinates 72° 55’ to 72° 54’ from latitude west and 13° 33’ to 13° 34’ south longitude. The climate in Abancay is 16.7 °C annual average temperature and 685 mm annual average rainfall [36]. The access to the Ampay glacier from the city of Abancay is by paved road and road, traveling 51 km to Karcatera, where it is possible to find informative signs, and then follow a 6-hour road to reach the base camp.
The Ampay glacier is part of the Apurímac river basin, on the Atlantic slope, sub-basin of the Sahuanay River, which plays an important role in the water supply to the ‘National Shrine of Ampay’.
The snow-capped mountain is located within the Ampay National Sanctuary, natural heritage of the Apurímac area, climate regulator and the only natural water reserve in the buffer zone of the sanctuary. The retreat of the glaciers of the Andes Mountains of Peru began in the mid-1980s [30]. The mass loss of the glaciers and the elevation is inversely related, the higher the altitude, the lower the loss of mass [20]. During the year 2017 in the Ampay glacier, 10 samplings have been carried out, time that allowed to appreciate the retreat of the Ampay glacier, especially the BC concentration, of the months of February to June 2017 (Figure 5) and the July to November (Figure 6).
In Figure 5 and Figure 6 capture of BC in the filters is displayed, showing the evolution by months, with the month of October being the one with the highest amount of BC. The concentration of dark particles in the filters is noticeable as time passes. This coincides with the culmination of the rainy season and the start of an unfortunate time, that of forest fires and pastures, which, according to the reports mentioned, are the main source of BC in tropical glaciers.
Time evolution of BC measurement
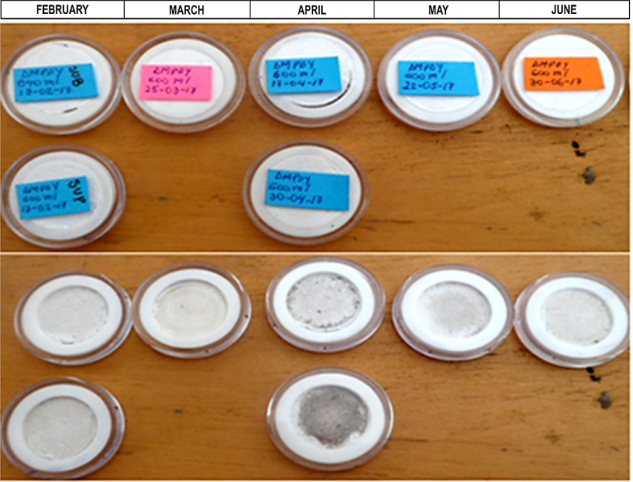
Accumulation of BC, in quartz filters
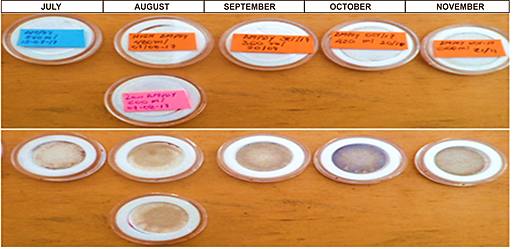
In Figure 4, Figure 5 and Figure 6, the obtained filters are exposed to the light of the LAHM instrument and the Arduino software, represented in the central column and whose unit is expressed in nano grams of BC per gram of snow.
These values are incorporated into another free simulation package, called SNICAR, which allows knowing the albedo of each filter, that is, the amount of light retained, with respect to the blank or clean filter, which is seen in the last Snow Albedo. In Table 1, and Figure 4, how the values obtained from the clean filter simulation, compared to those with BC, Figure 5 and Figure 6.
In the Table 2 and Figure 7, it is shown that the highest amount of BC captured with the quartz filters has been obtained in October with 65.224 nano grams of BC per gram of water (ng of BC/g of water) and the lowest amount has been in February with 20.941 ng of BC/g of water.
Calculation of BC with LAHM equipment and Arduino software
Months |
Value [ng of BC/g of water] |
Snow albedo (SNICAR) |
Difference** (Referent – month) |
Witness* |
0.00 |
*0.88614756 |
0 |
February |
20.94 |
0.88110042 |
0.0050 |
March |
22.86 |
0.88072866 |
0.0059 |
April |
26.98 |
0.87886762 |
0.0072 |
May |
27.76 |
0.87981164 |
0.0063 |
June |
28.65 |
0.87965477 |
0.0064 |
July |
30.88 |
0.87924904 |
0.0068 |
August |
35.97 |
0.87837684 |
0.0077 |
September |
51.59 |
0.87591600 |
0.0102 |
October |
65.22 |
0.87397921 |
0.0121 |
November |
62.86 |
0.87430507 |
0.0118 |
*Reference
Source: Data simulation with SNICAR, January 2018
BC ‒ LAHM
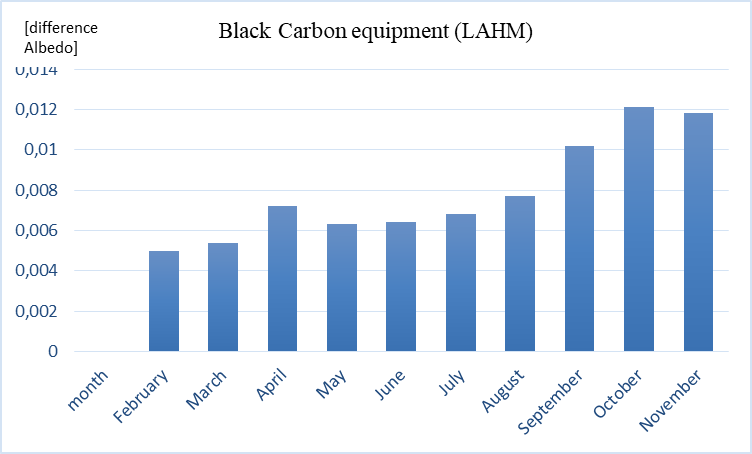
In Table 2, to calculate the accumulated energy of the month (kW/m2) the value of the daily solar energy has been multiplied by the number of days. For example, February: 5.4 × 28 days = 151.2 kW/m2, and so every month.
Carl Schmitt in the research carried out in Peru applying the LAHM method showed that after three years of evaluation the concentrations of effective BC range from: 2.0 ng of BC/g of water to almost 80 ng of BC/g of water. In the Ampay glacier, the values obtained after one year in 2017, were from 20,941 ng of BC/g of water to 65,224 ng of BC/g of water, and considered high, so it is necessary to continue carrying out similar studies in the study area to evaluate the evolution of BC.
It is necessary to know the existing radiation in the area and in the months of study. In this case, the historical data of the National Service of Meteorology and Hydology, where kWh/m2 is used, which allows the calculation of the energy absorbed by BC per month in fresh snow.
The energy absorbed by the BC goat in the Ampay glacier has higher amounts in the month of November with 8,952.92 J s/m2.
In the month of November in the surrounding areas and the buffer zone of the Ampay National Sanctuary where the snow is located, the villagers usually make burns of pastures and forests irresponsibly, being the hottest month and with total absence of rainfall what obliges farmers in their ancestral beliefs to carry out burning activities, increasing BC pollution. On the contrary, the month of February is the month with the highest annual rainfall, registering a 2,747.26 J s/m2 (Table 3 and Figure 8).
Energy absorbed by BC
Month of 2017 |
Total energy (TE) [kW/m2] |
TE × 3,600 [J s/m2] |
Difference |
Energy absorbed by BC [J s/m2] |
February |
151.2 |
5.4 |
0.0050 |
2,747.26 |
March |
196.0 |
7.0 |
0.0054 |
3,809.92 |
April |
173.6 |
6.2 |
0.0072 |
4,560.15 |
May |
170.8 |
6.1 |
0.0063 |
3,888.99 |
June |
142.8 |
5.1 |
0.0064 |
3,365.86 |
July |
154.0 |
5.5 |
0.0068 |
3,849.37 |
August |
123.2 |
4.4 |
0.0077 |
3,468.85 |
September |
168.0 |
6.0 |
0.0102 |
6,188.05 |
October |
201.6 |
7.2 |
0.0121 |
8,826.92 |
November |
210.0 |
7.5 |
0.0118 |
8,952.92 |
Energy absorbed by BC
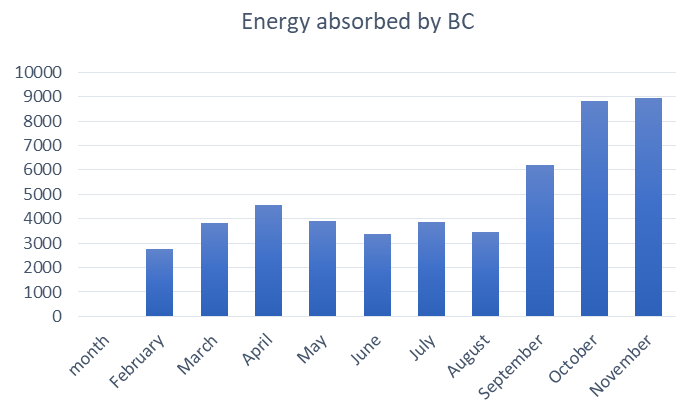
The peak months from September to November are the ones with the highest concentrations of BC. In September has been obtained 18.41 kg/m2 snow melted by BC, and October is has been obtained 26.27 kg/m2 and November has been obtained 26.65 kg/m2 (Table 4 and Figure 9).
Snow melted by the BC on the Ampay glacier
Month of 2017 |
Energy absorbed by BC [J s/m2] |
Fusion energy [J s/m2] |
Snow melted by the BC [kg/m2] |
February |
2,747,259.24 |
336,000 |
8.176366 |
March |
3,809,920.21 |
336,000 |
11.339048 |
April |
4,560,154.42 |
336,000 |
13.571888 |
May |
3,888,987.70 |
336,000 |
11.574368 |
June |
3,365,862.34 |
336,000 |
10.017447 |
July |
3,849,374.16 |
336,000 |
11.456470 |
August |
3,468,849.41 |
336,000 |
10.323956 |
September |
6,188,047.49 |
336,000 |
18.416808 |
October |
8,826,921.09 |
336,000 |
26.270598 |
November |
8,952,922.44 |
336,000 |
26.645602 |
Snow melted by BC during the year 2017
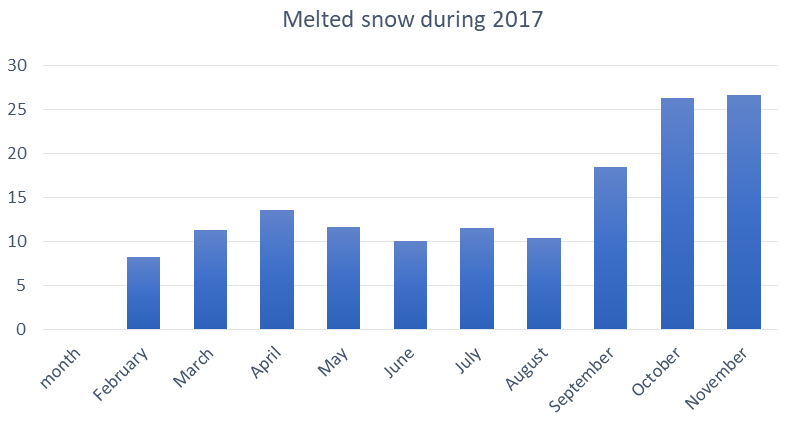
In these months the inhabitants of the surrounding and buffer zones of the Ampay National Sanctuary cause forest fires very frequently, showing these samples in Figure 5 and Figure 6.
The melted snow due to BC in the Ampay glacier has been calculated in kilograms per square meter as indicated in Table 3 and Figure 9. The month that less BC concentrations occur on the surface of the Ampay glacier is February because it is a month of rainfall in the study area (Figure 9).
BC, commonly known as soot, is made up of tiny solid particles. Its size can be less than the thickness of a human hair [1]. Specialists usually call it particulate material, which has a strong absorption capacity of solar energy and is produced during incomplete combustion [37].
The main objective has been studying the quantitative estimate of BC in the Ampay glacier, during 2017. It allowed determining the temporary concentration in the monitoring months from February to December. It is considered as possible sources of emission of this pollutant in the glacier of the Ampay National Sanctuary protected area which increases the importance of the study.
The calculation of the BC has been carried out with the LAHM equipment and Arduino software, obtaining the highest value of 65,224 ng of BC/g of water in October and the minimum value of 20,941 ng of BC/g of water in the month of February associated with rainfall. In the months with high temperatures, September, October and November, the results are associated by sources of emissions such as forest fires, burning of agricultural residues, pastures and the use of biomass for cooking.
Although there is only BC concentration values for this glacier, in the reports of the National Institute of Glaciers and Mountain Ecosystems [37], it can be seen that the BC estimates that in the Shallap and Vallunaraju glaciers of the White Mountain are above those found in Ampay, perhaps because Huaraz is a larger city and has near its mountains a great anthropic activity that would raise such measurements.
Given the altitude, the decrease in cover is particularly noticeable between 4,500-5,500 m, and changes in the last altitude are increasingly dominant over time and worldwide, reducing the coverage of glaciers in the area tropical in more than 60% [38].
The Ampay glacier is located at 5,235 meters, noting that the decrease of the glacier is imminent. The energy absorbed by BC in the Ampay glacier has been greater in November with 8,952.92 J s/m2 like in the month of February than with 2,747.26 J s/m2.
The change in coverage has been reduced to the average Oceanic Child Index for 6 periods between 1987 and 2016. The relationship shows a certain amount of dispersion, which has not yet been explained, but is still significant.
The amount of snow melted in February has been 8.17 kg/m2, this during the rainy season. In the months of May to November, the concentration of BC is ascending due to the fact that the grassland fire season also began, thus obtaining 26.65 kg/m2 of melted snow per m2 in the month of November.
Forest fires, grassland burning, pollution generated by the vehicle fleet, the burning of agricultural and pasture residues, the use of biomass (wood) for cooking and the use of fossil fuels in the Apurimac region are an important source of emissions. However, in the rest of the world, the largest emission of BC is the use of coal for power generation, heating and transportation [39].
Recent decreases in ice mass have been correlated with the increase in surface air temperature [40]. The main components of BC are: sulfates, nitrates, ammonia and sodium chloride. The main elements found when burning fossil fuels, when processing metals and incinerating wastes are: lead, cadmium, zinc, copper, nickel, vanadium, antimony [41]. By applying the method of analytical, it has been possible to demonstrate that the snow collected in several places of the Ampay glacier contains between 80 and 95% of BC particles that are absorbed by the particles in the snow [42]. The Center for Research on Climate Change and Environmental Management will collect snow samples for analysis on the Atomic Absorption Spectrometer, for the validation of BC values [30]. The collaborating scientists of this institution will seek to identify the sources of BC through chemical analysis [43]. The results obtained from the collection of samples of melted snow using the quartz filters have allowed the validation of BC values and will be references for other investigations because they are the first metadata to date.
The light absorbing particles in the Ampay glacier indicate that there is a significant impact on the region. The levels of BC vary substantially according to the season, increasing its highest values at the end of the dry season, which coincides with the burning of grassland and biomass for cooking.
While reducing the rise in temperature due to climate change is a global challenge, reducing local sources of particles that absorb light is a local challenge. The reduction of light absorbing particles produced in the department of Apurimac could prolong the life of the Ampay glacier and guarantee a permanent source of natural water for agriculture and human consumption.
The energy absorbed by the BC in the Ampay glacier has been greater in the month of November with 8,952.92 J s/m2 unlike in the month of February than with 2,747.26 J s/m2.
The temporary variation of BC in the Ampay glacier has been considerable, especially in the winter months when temperatures rise (in the Andes of southern Peru) and it does not rain, this absence is prolonged for weeks and months causing the hand of man in many cases forest fires, grassland and biomass for cooking.
The month of April, reports a slight increase in pollutants with the presence of precipitation and snow, the amount of snow melted by BC action has been 13.57 kg/m2.
The other months increase their value considerably, being November the most polluted month, with a value of 26.65 kg/m2, almost 7.0 kg/m2 of snow become water.
By applying the method of analytical induction, it has been possible to demonstrate that the snow collected in several places of the Ampay glacier contains between 80 and 95% of BC particles that are absorbed by the particles in the snow. Between 8.0 L and 27.0 L of water are lost every month due to the action of BC during the dry season, which leads to an additional loss of snow from the Ampay glacier of at least 20 cm per year.
In the Ampay glacier, the values obtained after the year 2017 were from 20,941 ng of BC/g of water to 65,224 ng of BC/g of water, considered high compared to the data obtained by Carl Schmitt applying the LAHM method, for so it is necessary to continue carrying out similar studies in the study area to evaluate the evolution of carbon.
Climate change and tropical Andean glaciers: Past, present and future ,Earth-Science Reviews , Vol. 89 (3-4),pp 79-96 , 2008, https://doi.org/https://doi.org/10.1016/j.earscirev.2008.04.002
, Climate change threats to environment in the tropical Andes: glaciers and water resources ,Regional Environmental Change , Vol. 11 ,pp 179-187 , 2011, https://doi.org/https://doi.org/10.1007/s10113-010-0177-6
, Glacier inventory: Cordillera Blanca ,Glaciology and Water Resources Unit National Water Authority , 2012
, - Report to Congress on Black Carbon , 2012
Black Carbon: A Science/Policy Primer, Pew Center on Global Climate Change ,PEW CENTER ON GLOBAL CLIMATE CHANGE , 2009
, Climate response to regional radiative forcing during the twentieth century ,Nature Geoscience volume , Vol. 2 ,pp 294-300 , 2009, https://doi.org/https://doi.org/10.1038/NGEO473
, Impact of California’s air pollution laws on black carbon and their implications for direct radiative forcing ,Atmospheric Environment , Vol. 45 (5),pp 1162-1167 , 2011, https://doi.org/https://doi.org/10.1016/j.atmosenv.2010.10.054
, Climate Change: The Physical Science Basis, Contribution of Working Group I to the Fourth Assessment Report of the Intergovernmental Panel on Climate Change ,Intergovernmental Panel on Climate Change (IPCC) ,pp 996 , 2007
, Quantitative estimation of black carbon in the glacier ampay-apurimac ,Journal of Sustainable Development of Energy, Water and Environment Systems , 2020, https://doi.org/10.13044/j.sdewes.d8.0342
, Ice Sheet Mass Loss Caused by Dust and Black Carbon Accumulation ,The Cryosphere , Vol. 9 (2),pp 1845-1856 , 2015, https://doi.org/https://doi.org/10.5194/tc-9-1845-2015
, A Model for the Spectral Albedo of Snow ,Journal of the Atmospheric Sciences , Vol. 37 ,pp 2712-2733 , 1980, https://doi.org/https://doi.org/10.1175/1520-0469(1980)037<2712:AMFTSA\>2.0.CO;2
, Bounding the role of black carbon in the climate system: A scientific assessment ,JOURNAL OF GEOPHYSICAL RESEARCH: ATMOSPHERES , Vol. 118 ,pp 5380–5552 , 2013, https://doi.org/https://doi.org/10.1002/jgrd.50171
, Global atmospheric impacts of residential fuels ,Energy for Sustainable Development , Vol. 8 (3),pp 20-32 , 2004, https://doi.org/https://doi.org/10.1016/S0973-0826(08)60464-0
, Can Reducing Black Carbon Emissions Counteract Global Warming? ,Environmental Science and Technology , Vol. 39 (16),pp 5921-5926 , 2005, https://doi.org/doi.org/10.1021/es0480421
, Public Health Benefits of Strategies to Reduce Greenhouse-Gas Emissions: Urban Land Transport ,The Lancet , Vol. 374 (9705),pp 1930-1943 , 2009, https://doi.org/https://doi.org/10.1016/S0140-6736(09)61714-1
, Public Health Benefits of Strategies to Reduce Greenhouse-Gas Emissions: Household Energy ,The Lancet , Vol. 374 (9705),pp 1917-1929 , 2009, https://doi.org/https://doi.org/10.1016/S0140-6736(09)61713-X
, Adoption and Sustained Use of Improved Cook-Stoves ,Energ. Policy , Vol. 39 (12),pp 7557-7566 , 2011, https://doi.org/https://doi.org/10.1016/j.enpol.2011.03.028
, - National Air Quality Report, 2012, http://www.minam.gob.pe/wp-content/uploads/2016/07/Informe-Nacional%0A-de-Calidad-del-Aire-2013-2014.pdf
A Review of the Modern Fluctuations of Tropical Glaciers ,Global and Planetary Change , Vol. 22 (1-4),pp 93-103 , 1999, https://doi.org/https://doi.org/10.1016/S0921-8181(99)00028-4
, Current state of glaciers in the tropical Andes: a multi-century perspective on glacier evolution and climate change ,The Cryosphere , Vol. 7 (1),pp 81-102 , 2013, https://doi.org/https://doi.org/10.5194/tc-7-81-2013
, Threats to Water Supplies in The Tropical Andes ,Science , Vol. 312 ,pp 1755-1756 , 2006, https://doi.org/https://doi.org/10.1126/science.1128087
, Emissions from forest fires near Mexico City ,Atmospheric Chemistry and Physics , Vol. 7 (21),pp 5569-5584 , 2007, https://doi.org/https://doi.org/10.5194/acp-7-5569-2007
, Estimated Global Mortality Attributable to Smoke from Landscape Fires ,Environ. Health Perspect , Vol. 120 (5),pp 695-701 , 2012, https://doi.org/https://doi.org/10.1289/ehp.1104422
, Global Pyrogeography: The Current and Future Distribution of Wildfire ,PLoS One , Vol. 4 (4), 2009, https://doi.org/https://doi.org/10.1371/journal.pone.0005102
, Mega-fires, tipping points and ecosystem services: Managing forests and woodlands in an uncertain future ,Forest Ecology and Management , Vol. 294 ,pp 250-261 , 2013, https://doi.org/https://doi.org/10.1016/j.foreco.2012.11.039
, End of the Little Ice Age in the Alps Forced by Industrial Black Carbon ,P. Natl. Acad. Sci , Vol. 110 (38),pp 15216-15221 , 2013, https://doi.org/https://doi.org/10.1073/pnas.1302570110
, An Approach to a Black Carbon Emission Inventory for Mexico by Two Methods ,Sci. Total Environ , Vol. 479-480 ,pp 181-188 , 2014, https://doi.org/https://doi.org/10.1016/j.scitotenv.2014.01.064
, Measurements of light-absorbing particles on the glaciers in the Cordillera Blanca, Peru ,The Cryosphere , (9),pp 331-340 , 2015, https://doi.org/https://doi.org/10.5194/tc-9-331-2015
, Global and Regional Climate Changes Due to Black Carbon ,Nature Geoscience , Vol. 1 ,pp 221-227 , 2008, https://doi.org/https://doi.org/10.1038/ngeo156
, 20th-Century Industrial Black Carbon Emissions Altered Arctic Climate Forcing ,Science , Vol. 317 (5843),pp 1381-1384 , 2007, https://doi.org/https://doi.org/10.1126/science.1144856
, Global Warming in the Twenty-First Century: An Alternative Scenario ,P. Natl. Acad. Sci , Vol. 97 (18),pp 9875-9880 , 2000, https://doi.org/https://doi.org/10.1073/pnas.170278997
, Black Carbon Particulate Matter Emission Factors for Buoyancy Driven Associated Gas Flares ,Journal of the Air & Waste Management Association , Vol. 62 (3),pp 307-321 , 2012, https://doi.org/https://doi.org/10.1080/10473289.2011.650040
, Present-Day Climate Forcing and Response from Black Carbon in Snow ,Journal of Geophysical Research: Atmospheres , Vol. 112 (D11), 2007, https://doi.org/https://doi.org/10.1029/2006JD008003
, Springtime Warming and Reduced Snow Cover from Carbonaceous Particles ,Atmospheric Chemistry and Physics , Vol. 9 (7),pp 2481-2497 , 2009, https://doi.org/https://doi.org/10.5194/acp-9-2481-2009
, Rapid Calculation of Radiative Heating Rates and Photodissociation Rates in Inhomogeneous Multiple Scattering Atmospheres ,Journal of Geophysical Research: Atmospheres , Vol. 94 (D13),pp 16287-16301 , 1989, https://doi.org/https://doi.org/10.1029/JD094iD13p16287
, Potential Phytoremediator of Native Species in Soils Contaminated by Heavy Metals in the Garbage Dump Quitasol-Imponeda Abancay ,J. Sustain. Dev. Energy Water Environ. Syst , Vol. 7 (4),pp 584-600 , 2019, https://doi.org/https://doi.org/10.13044/j.sdewes.d7.0261
, Glacier and Climatic Trends Derived from Multiple Data Sources in the Vilcanota Mountain Range, Southern Andes of Peru ,The Cryosphere , Vol. 7 ,pp 103-118 , 2013, https://doi.org/https://doi.org/10.5194/tc-7-103-2013
, - , Impact of Black Carbon on the Glaciers of the White Mountain Range, May 23, 2018
Prototype Land-Cover Mapping of the Huascaran Biosphere Reserve (Peru) Using a Digital Elevation Model, and the NDSI and NDVI Indices ,Journal of Applied Remote Sensing , Vol. 3 (1), 2009, https://doi.org/https://doi.org/10.1117/1.3106599
, Observations: Cryosphere, in: Climate Change: The Physical Science Basis. Contribution of Working Group I to the Fifth Assessment Report of the Intergovernmental Panel on Climate Change ,Cambridge University Press ,pp 317-382 , 2013
, Characterization of Atmospheric Trace Elements on PM2.5 Particulate Matter Over the New York – New Jersey Harbor Estuary ,Atmospheric Environment , Vol. 36 (6),pp 1077-1086 , 2002, https://doi.org/https://doi.org/10.1016/S1352-2310(01)00381-8
, Black Carbon and its Effects on the Climate, Balance and Perspectives ,Factor CO2 ,pp 1-52 , 2014
, Sources of Light-Absorbing Aerosol in Arctic Snow and Their Seasonal Variation ,Atmos. Chem. Phys , Vol. 10 ,pp 10923-10938 , 2010, https://doi.org/https://doi.org/10.5194/acp-10-10923-2010
,